Bioactive vs. Biocompatible: What’s the Difference?
Posted Dec 1, 2021
on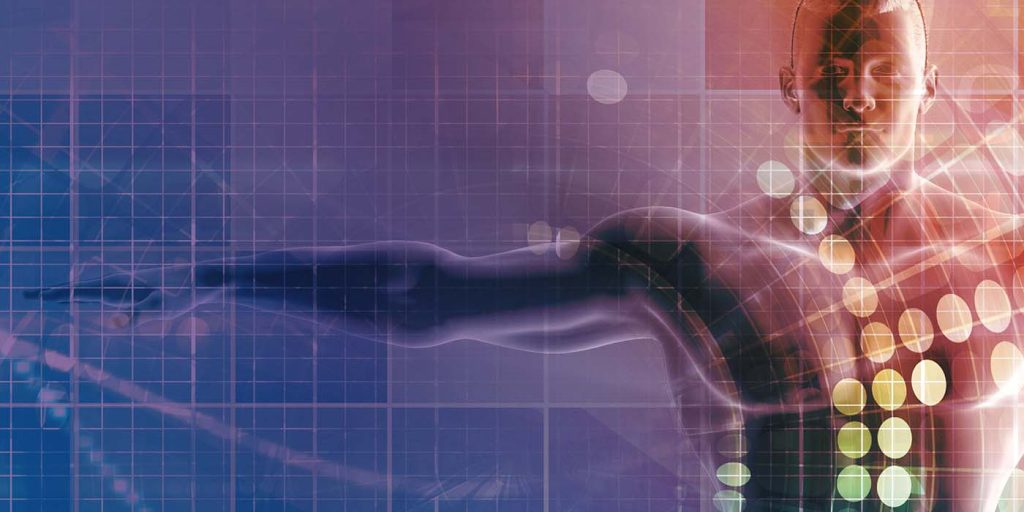
The study of biomaterials — substances that are engineered to interact with biological systems for medical purposes — is a relatively new field, but one that has already produced a number of valuable discoveries and treatments.
Over the last few decades, the field has grown rapidly; and, with it, the list of new terms used to describe biomedical devices and materials. In this article, we take a look at two of the most common adjectives in the field, bioactive and biocompatible, and how they are used to describe a range of highly specialized materials.
Essentially, the term biocompatible is used to describe any material which is well-tolerated by the biological system it comes into contact with. At a minimum, most biocompatible materials are chemically inert. In practice, biocompatibility for a given application may depend on any number of other characteristics such as mechanical strength, elastic modulus, or adhesion with the surrounding cellular matrix. It is important to note that biocompatibility is application-specific: a material that is deemed biocompatible in one specific use case may not be biocompatible in another.
Bioactive materials go a step further – these are materials that produce some kind of local physiological response, typically through physical or chemical action. A common example of a bioactive material is bioactive glass: typically silicate-based glass-ceramic materials that are often degradable within the body. Used for bone repair, ion exchange at the surface of bioactive glass leads to the formation of bone-like hydroxyapatite, around which natural bone will readily grow.1 In this way, bioactive glass can actively stimulate the regeneration of bone within a patient.
The term “bioactive” is the opposite of “bioinert”, a word that refers to materials that don’t produce a physiological reaction.
Definitions and Distinctions
If we had to pick “official” definitions of “biocompatible” and “bioactive”, it would probably be those offered by The International Union of Pure and Applied Chemistry (IUPAC), a world authority on standardized scientific nomenclature.2
Bioactive: “Qualifier for a substance which provokes any response from a living system”. IUPAC also notes that the term is often used positively, i.e., to reflect a beneficial change.
Biocompatibility: “The ability to be in contact with a living system without producing an adverse effect.”
These definitions seem simple enough, but there are some subtleties that often cause confusion. These can be cleared up by thinking about how we apply the terms to a very familiar medical device: a contact lens.
Biocompatibility without Bioactivity
Modern contact lenses are made from soft, inert synthetic polymers known as silicone hydrogels. Silicone hydrogel contact lenses are wettable, permeable to oxygen, and cause minimal irritation to the tissues of the eye. We can confidently say, then, that modern contact lenses are highly biocompatible when used properly.
But is a contact lens bioactive? Although a contact lens modifies the light as it enters the eye (resulting in improved vision), the contact lens does not produce a local physiological response with the eye tissue. Thus, we can’t refer to an ordinary contact lens as bioactive.
Note also that “biocompatible” is typically used only in reference to materials, while the term “bioactive” is used to refer to materials or drugs. So, a drug that is tolerated well and without negative side effects would not typically be described as “biocompatible” even though it meets the IUPAC definition.
Mechanisms of Bioactivity
The mechanisms by which bioactivity can occur are numerous and often complex.3 Attempts to engineer bioactive materials often involve emulating intra-cellular signaling, using coatings of proteins or peptides to modulate cell interactions.
There are also many bioactive materials – largely within the category of bioactive glasses and ceramics – which produce a local physiological response through the exchange of simple ions such as silver, fluoride, and calcium.4 These “therapeutic ions” can activate, inhibit or enhance a huge array of cellular pathways, resulting in effects such as antibacterial activity, and stimulation of bone and blood vessel formation.
As research continues and scientists learn more about how different materials can influence cellular processes, we can expect more biocompatible and bioactive materials to find their way into mainstream medical treatment.
Mo-Sci has extensive experience in the manufacture of bioactive glasses. We produce standard compositions, such as 45S5, S53P4, and 13-93, and can also research, develop, and manufacture glass that is customized to fit your application. Contact us to see how we can help with your next product.
References and Further Reading
- Ferraris, S. et al. Bioactive materials: In vitro investigation of different mechanisms of hydroxyapatite precipitation. Acta Biomaterialia 102, 468–480 (2020).
- Vert, M. et al. Terminology for biorelated polymers and applications (IUPAC Recommendations 2012). Pure and Applied Chemistry 84, 377–410 (2012).
- Meyers, S. R. & Grinstaff, M. W. Biocompatible and Bioactive Surface Modifications for Prolonged In Vivo Efficacy. Chem. Rev. 112, 1615–1632 (2012).
- Baino, F., Hamzehlou, S. & Kargozar, S. Bioactive Glasses: Where Are We and Where Are We Going? JFB 9, 25 (2018).